Exploration & Innovation: Geoscientists Push the Frontiers of Unconventional Oil and Gas
April 12, 2008
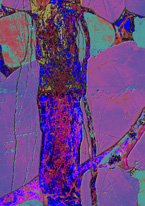
As petroleum supplies tighten, crude oil prices remain high, and energy security continues to concern U.S. politicians, the world is increasingly turning its attention to unconventional oil and gas.
Energy analysts now routinely accept that the world’s unconventional hydrocarbons, such as gas hydrates, tight gas sandstones, and oil and gas shales, hold more fuel than undiscovered conventional energy sources. While the potential of unconventional oil and gas has long been acknowledged, a host of challenges have dampened expectations.
Today, this seems set to change. Unconventional hydrocarbons currently account for less than 15 percent of the world’s global oil production of about 70 million barrels per day, according to energy consulting firm Wood Mackenzie. But by 2025, predicts Wood Mackenzie, unconventional oil will supply more than 20 percent of global demand. Unconventional gas will be even more important, especially in the United States, where it already accounts for more than 40 percent of the natural gas supply, according to the U.S. Department of Energy.
Coal Gasification
Regains Spotlight Coal is sometimes referred to as a dirty fuel because of pollutants released when it burns. Gasification might help turn coal into an eco-friendly fuel for the future.
|
Until recently, the general consensus was that unconventional oil and gas were too expensive and technically difficult to pursue. But skyrocketing fuel prices and major technology advances have changed that outlook dramatically.
“We now see that there is enormous potential in unconventional oil and gas,” said William Ambrose, a research scientist at the Jackson School’s Bureau of Economic Geology. As president of the Energy Minerals Division of the American Association of Petroleum Geologists in 2006-2007, a professional organization whose mission encompasses advancing the science of unconventional hydrocarbons, Ambrose is in a position to know. He is part of a core of researchers at the Jackson School, principally in the Bureau of Economic Geology, working to advance geoscience knowledge of unconventional resources.
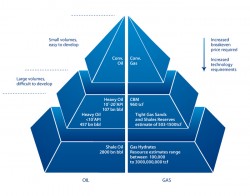
Some unconventional supplies are already fueling our homes. “What were once considered these avant-garde resources that nobody dared to approach are now suddenly well sought-after commodities in the industry,” said Ambrose.
Energy security concerns further heighten interest in unconventional resources. Unlike conventional hydrocarbons, unconventionals are well distributed in the United States and some of its closest allies, like Canada. Pursuing unconventional resources, the political argument goes, can reduce American dependence on oil from more volatile regions of the world.
Despite a rosier forecast for unconventionals, however, they still face many obstacles. While fuel from traditional reservoirs doesn’t need much persuasion to flow to the well, coaxing oil and gas out of unconventional hideouts can be a huge headache. Not only are the fuels locked away tightly but they also accumulate in spots that are often hard to get to. In fact, the largest amount of unconventional gas may be sitting in one of the least accessible places on the planet: the ocean floor.
Gas on the Rocks
Squeezed together under megatons of water, methane litters the seafloor and the mud and rock beneath it in the form of hydrates—ice-like deposits of gas and water. Hydrates occur when water molecules form crystalline structures leaving cavities which can be occupied by a single gas molecule. The structure enclosing the gas is known as a hydrate cage.
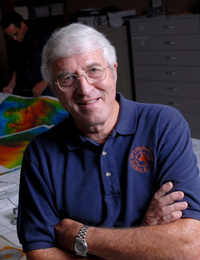
Because of the crushing pressures and chilling temperatures at the bottom of the ocean, gas trapped in hydrate cages is highly concentrated. “The energy density of hydrates is 42 percent that of liquefied natural gas,” said Bob Hardage, senior research scientist at the Bureau of Economic Geology. “LNG is the highest energy concentration of natural gases that man can make, and hydrate is not far behind LNG technology in terms of efficiency of energy concentration.” If captured, these hydrate formations could be abundant reservoirs of fuel. But while researchers have had some success recovering hydrates from permafrost regions in the Arctic, marine sites remain a challenge.
“Whether or not you can actually produce gas from seafloor hydrates is still a big question,” said Hardage. The technological challenges are formidable and scientists have raised safety and environmental concerns.
There are several ways to release the gas molecules from their hydrate cages that include decreasing the pressure to liberate the gas or increasing the temperature to melt the deposits. But one needs to know that the seafloor is stable before pursuing either of those options, said Hardage. “If you reduce the pressure in an unstable area, the seafloor might slump and you could lose a good chunk of shallow strata.” That could release massive amounts of methane into the water column and eventually into the atmosphere. And because methane is a greenhouse gas about 20 times as potent as carbon dioxide, such an event could contribute significantly to global warming.
Institute Pioneers
Research on Hydrates Institute for Geophysics researchers pioneered the earliest efforts at detecting gas hydrates, advanced our understanding of their vertical orientation in venting systems and are now addressing critical questions on their potential release due to warming oceans. |
Even though scientists don’t know exactly how much natural gas is bound in hydrates, most agree the quantities are enormous. “There is a tantalizing possibility that the amount of energy available in hydrates exceeds that of conventional gas resources by several factors,” said Hardage. But the exact numbers are still contentious, partly because it’s difficult to image the deepwater deposits. Determining amount and distribution of methane hydrates near the ocean floor is currently the primary research focus in the field, said Hardage.
He and his team are working with multi-component seismic data to create detailed profiles of near-seafloor strata beneath the Green Canyon area in the Gulf of Mexico where heavier thermogenic gases (ethane and propane) fill some of the hydrate cages and cause the hydrate to be stable over a wider range of pressure and temperature than is pure-methane hydrate. By sending sound waves through the ground and measuring how fast they travel, the team can gain clues about the composition of the sediments, such as potential hydrates content. Rather than the commonly used compression waves, Hardage and his team use shear waves, which provide better resolution. “We can see far more geological detail through the hydrate systems than what can be seen with compression waves,” said Hardage.
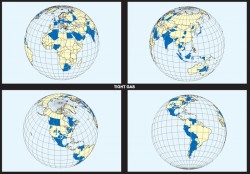
Based on their data, Hardage and his team have developed rock physics models that relate seismic wave velocities to different percentages of hydrate concentrations in various mixtures of sand, clay, and brine.
Deeper Resources
While gas hydrates are still in the experimental phase, commercial production of several other unconventional gas resources is already in full swing. According to the U.S. Department of Energy, coalbed methane, shale gas, and tight gas currently account for more than 40 percent of U.S. gas production. “We have basically gone through the resources that are easy to locate and produce and now we have turned to the next category,” said Eric Potter, associate director for energy programs at the Bureau of Economic Geology. “And even though this gas is dispersed and expensive, it’s still a lot more realistic to produce than alternative forms of energy like wind or solar.”
A major unconventional gas resource is tight sandstone—rock with low permeability and porosity. Such formations develop when geological processes such as compaction and cementation close the large pores of the rocks that house conventional deposits. Gas can easily flow through the large pore throats that permeate regular sandstones but in tight sandstones it is trapped. Producers have to employ special treatments to extract it. One option is hydraulic fracturing—a technique in which mixtures of water and sand or ceramic beads are pumped into the rock formation. The pressure eventually cracks the rocks, allowing the gas to flow to the well.
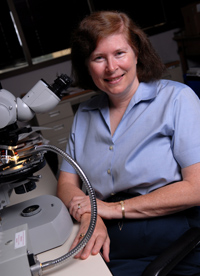
But all tight sandstones are not alike, said Shirley Dutton, senior research scientist at the Bureau of Economic Geology. “Some areas produce better than others (sweet spots), and it’s still poorly understood what controls the production,” said Dutton. Geoscientists around the world are trying to find answers to that question. One factor is how the sand was originally deposited. Depositional history determines the regional distribution, geometry, and texture of the reservoir sandstones. However, Dutton noted, production characteristics of tight gas sandstones are in large part controlled by the diagenesis, or chemical and physical changes, that the sediment has undergone after deposition. Extensive cementation is commonly the reason for low permeability. Finally, natural fractures can be locally abundant in tight gas sandstones and may play a key role in production. Open natural fractures provide pathways for fluid movement and affect the way that hydraulic fractures grow.
Dutton and her colleagues are using seismic data and rock samples to study deeply buried sandstones (>15,000 ft) below the shallow water of the Texas Gulf coast. A lot of the conventional oil and gas fields in this area are declining, and there is now a move to evaluate the kinds of reservoirs that lie beneath these depleted fields. High temperatures and pressures make drilling 20,000 or 30,000 feet deep an especially risky proposition, making it all the more important to devise methods to gauge the economic viability of resource plays at these depths. “For example, we are investigating whether there is a reservoir basement, so we could say, ‘If you get beyond a certain temperature or depth, an economic rate of production becomes unlikely,’” said Dutton.
Going to the Source
Fossil fuels don’t always manage to escape from the source rock. That’s why shales—the ancient oil and gas factories that generated the fuels over millions of years—are another popular unconventional resource of natural gas. According to Ambrose the U.S. gas resource from shale beds ranges from 500 to 780 trillion cubic feet, though with current technologies only about one tenth of that can be recovered, he added.
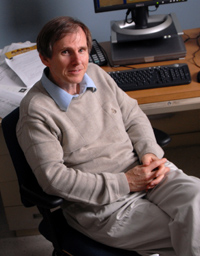
As producers in the famous Barnett Shale have taught the world, fractures are essential for gas production from shales. “The key to the Barnett Shale formation is being able to fracture the formation and to maintain the flow,” said Ambrose. The shale’s susceptibility to artificial fractures varies greatly and geoscientists are currently trying to find out what makes some shales break easier than others.
Variations in the mineral content of a rock and the particle types it contains all contribute to its mechanical strength, said Steve Ruppel, senior research scientist at the Bureau of Economic Geology. “The word shale encompasses a really broad range of mineralogic and sedimentary properties,” said Ruppel. He and his team try to determine how their composition varies across different areas, such as the Fort Worth Basin and the Permian Basin.
The recent economic success of shale gas production has sparked a lot of research interest in shale successions, said Ruppel. “In the past there was relatively little economic value in shales so interest in studying them was limited. Now these rocks are receiving a lot of attention because we need to know their mineral composition and strength as well as the distribution of these properties,” he said.
Even though shale gas production is booming, several problems have yet to be addressed. “Water is a huge issue,” said Ruppel. Not only are producers competing for surface water resources required for the drilling process but water contaminated with hazardous chemicals and elements generated in the production process needs to be disposed of. Companies currently drilling in the Barnett Shale, for example, re-inject these waters deep into the geologic column. But water treatment procedures will eventually have to be developed, Ruppel said: “Otherwise you will have to truck the water off to a disposal facility. That would drive up production costs.”
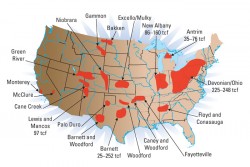
Still other environmental considerations play a role as well. Production of unconventional oil and gas resources has an intensive impact on the landscape compared to old-style exploration and production, said Potter. Unconventional resources require closely-spaced wells covering large areas—often multiple counties in a given play. “It’s essential to minimize the impact on wildlife and on residents.”
While commercial shale gas production has taken off, oil shales are an entirely different story. Attempts to develop a shale oil industry in the U.S. go back almost a century but the process is still too costly to be economical. “With shale gas all you have to do is open up a pathway and the buoyancy of the gas will bring it to the surface,” said Ambrose. But that’s not enough to coax oil out of shale. “Instead, you have to heat the rocks to about 450 degrees C (842 degrees F) to liquefy the oil.” That in itself is currently not economical. And water consumption plays a big role in oil shale production as well, adding substantially to the cost, Ambrose said. “But the fascinating element of all of this is that the technology keeps improving, so you never know what might happen in the future.”
by Nicole Branan
For more information about the Jackson School contact J.B. Bird at jbird@jsg.utexas.edu, 512-232-9623.