Roland Bürgmann: Geodesists Refine Plate Tectonic Tools
October 13, 2016
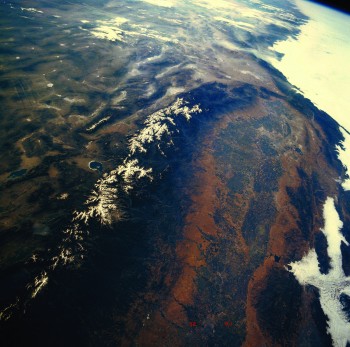
By Barbra Rodriguez
Geodynamics experts have used GPS to detect horizontal movement of tectonic plates for decades. In an October 2015 talk at the Jackson School, Roland Bürgmann, director of the Active Tectonics Research Group at the University of California, Berkeley, revealed how an enhanced GPS network and related aerial observations have allowed vertical changes in crustal motion, including non-tectonic sources of deformation, to finally be investigated.
His talk, made possible by the Jackson School’s Judd H. and Cynthia S. Oualline Centennial Lectureship in Geological Sciences, focused on a section of the San Andreas Fault running roughly parallel to and east of California’s San
Joaquin Valley. This heavily farmed valley is bounded by a mountainous coast range to the west and the Sierra Nevada mountains to the east.
Thanks to the Plate Boundary Observatory project, Bürgmann noted, more than 1,000 GPS stations now exist in the actively deforming western coast of California. The sheer number of GPS stations capturing data as often as every second has allowed scientists to capture precise 3-D measurements of tectonic plate motion.
Bürgmann reviewed vertical deformation studies and what is known about the effect of removing large masses of water during uplift or subsidence of the Earth’s crust near the San Andreas Fault. Bürgmann’s group has collaborated with researchers from the University of Nevada, Reno, who were the first to indicate that vertical—not horizontal—deformation was occurring.
In particular,the Reno researchers’ study in 2012 suggested that the Sierra Nevada had undergone vertical uplift of 1 to 2 millimeters annually in recent years, with the mass loss of sediment from the Sierras due to erosion being among potential causes.
Another study that piqued Bürgmann’s curiosity was about whether groundwater removal from the San Joaquin Valley for agricultural purposes could factor into this vertical uplift; that 2011 study led by University of California, Irvine, researchers used measurements from the GRACE (Gravity Recovery and Climate Experiment) satellite to indicate that the Central Valley had lost enough water between 2003 and 2007 to fill Lake Mead.
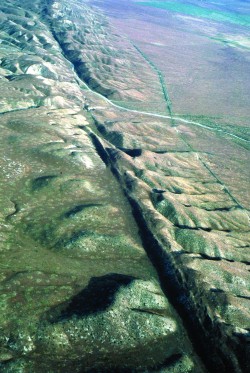
Carrizo Plain, California.
By combining GPS station data with that derived from InSAR (interferometric synthetic aperture radar), Bürgmann and colleagues studied water load impacts on vertical deformation throughout the Central Valley, Sierras and the coast range. InSAR can survey areas unreachable by GPS because it doesn’t require installing land-based equipment.
It instead maps deformation by using a satellite or aircraft radar system to detect reflected electromagnetic waves and the phase change of the return signal between flyovers. The researchers detected general uplift of coastal and Sierra Nevada locations from measurements taken for three or more years, whereas Central Valley stations subsided as much as 3 centimeters annually.
“That’s as fast as the San Andreas Fault moves laterally,” Bürgmann said, adding that seasonal patterns of deformation occurred.
For instance, although stations in the Central Valley generally subsided over time, some underwent uplift during the wet winter and early spring. The researchers reviewed data to decipher whether San Joaquin Valley water load changes were related to irrigation rather than shifting tectonic plates causing some areas to undergo crustal rebound and uplift. Hydrological processes turned out to underpin vertical deformation changes in general, he said.
“It’s not the highest (Sierra) mountains that move up the fastest, but it’s actually the low-lying areas next to the valley … because they are closest to the unloading of the water.” He noted that for the Coast Range and Sierras overall, tectonic factors held sway.
Bürgmann also discussed early studies on large-scale water movements as a force behind earthquakes. Removing a water load equivalent to the mass of Lake Mead (with an overburden of about 1 kilopascal of pressure) likely does not register long term on larger faults, he said, because geologic forces produce much higher stress overall (up to hundreds of megapascals in pressure) and annual fault-loading rate (up to 50 kilopascals). To see if water load changes or other factors influence smaller earthquakes, Bürgmann and colleagues considered a well-studied area around Parkfield, California.
They reviewed 30 years of data on three different types of fault stresses (normal, shear and Coulomb) and other factors for 4,000-plus earthquakes of 2.5 magnitude or above. Based on data, seasonal changes in seismicity were identified that complicated underpinnings, with water load a likely factor in some cases, along with factors such as fault orientation.
“You really have to consider the individual orientation of faults to ultimately make out how they are being affected by these very small stress changes,” he noted of this work in progress. Given the many smaller earthquakes and their annual faultloading rates, Bürgmann said that seasonal water load changes near some faults could “be enough to break the camel’s back.”
Back to the Newsletter