Morphodynamics Lab: Modeling Turbidity Currents
April 18, 2009
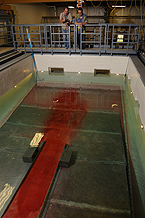
Pick your favorite river that flows to the sea, say the Mississippi or the Amazon. Now go to the mouth of that river and dive below the waves and you’ll notice a curious thing: the river keeps on going, skirting the bottom of the ocean. You can tell it from the surrounding water by all the sediment it’s carrying. All the sand, dirt, rocks, and organic matter it’s dragging along makes it denser than the surrounding sea water, so it sinks to the bottom and snakes along scouring channels, tributaries and canyons like a river on land. It looks a bit like an avalanche of snow tumbling down a mountainside. This is called a turbidity current. You can also find them on smaller scales in lakes and in reservoirs behind dams.
Scientists want to understand the fundamental forces at work in turbidity currents—what dictates their flow paths, what keeps them going over miles and miles of sea floor, how they reshape the seascape, and how the ancient channels they leave behind reflect past climate and flow rates. Oil and gas companies are also interested in better understanding them because the currents deposit organic matter that over geologic time gets buried, compressed and transformed into hydrocarbons.
Researchers have had a difficult time trying to recreate some aspects of these currents in the lab. David Mohrig and Jim Buttles, researchers at the Jackson School of Geosciences, know as well as anyone. They spent several years attempting it with large water tanks at their former academic home, the Massachusetts Institute of Technology (MIT). They would pour a layer of sediment in the bottom of a tank to mimic a sloping sea floor and add a layer of water to mimic the sea. Then at the shallow end, they would inject water containing sediment, which would form a turbidity current. They would then sit back and let it intermittently flow over the sediment bed for several days or even weeks. The trouble was, the currents wouldn’t cut channels in the sediment. The best they could do was first make artificial channels in the sediment and then watch how they evolved.
Mohrig, now an associate professor in the Jackson School, came to Austin in 2006. Buttles, now a research engineer and scientist associate in the school, followed in 2007. Together, they’ve built a new, larger tank. Housed in a warehouse on the J.J. Pickle Research Campus, it’s about the size of a regular back yard swimming pool with concrete walls one foot thick and an observation deck. They call it the UT Morphodynamics Lab (UTML).
The bottom of the tank features a ramp tilted up 10 degrees to simulate a continental slope, the place where a continent meets the deep seafloor. A typical continental slope would have a slope of about 4 degrees, but to get the physics right on a much smaller scale, a computer model of the system indicated that a steeper slope would be required. The sediment that forms the “sea floor” in the tank is a mix of plastic grains and clay particles that together have the right particle size, cohesiveness and density to mimic real sediments.
Front Row Seat
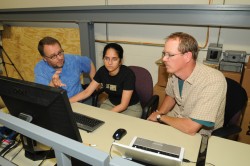
The researchers have an array of instruments to measure what’s happening as an experiment unfolds. Rows of digital cameras mounted above the tank and along the sides capture panoramic snapshots from multiple angles simultaneously. A laser altimeter scans back and forth over the tank measuring the topography of the sediment surface to within 100 microns vertically. An acoustic Doppler velocimeter inserted into the water measures the flow velocity and direction at specific points. An acoustic Doppler profiler measures discrete flow at many points within a column of water, providing a vertical profile. And an array of aluminum siphons sample water and sediment at specific depths and X,Y coordinates. The samples can be tested for fluid density, particle size and other characteristics.
But the observations don’t end when the currents stop flowing. At the end of an experiment, the researchers can drain the water and do a couple of other interesting investigations with the sediment. They extract “cold cores” by chilling a metal tube with liquid nitrogen, sticking it into the sediment and pulling it out. The core is a frozen ring of water and sediment that sticks to the outside of the tube. That hollow ring can be pulled off, sliced and analyzed to evaluate the stratigraphy of sediments at that spot. Researchers can also slice through the layers of sediment on the tank floor along one axis and evaluate the stratigraphy of the cross section.
This world-class facility provides opportunities for graduate students to try their hand at advanced research. Anjali Fernandes is a doctoral student in geological sciences working under the supervision of Mohrig and Ron Steel, professor and Davis Centennial Chair. She is trying to understand how turbidity currents create channels and how they later modify them to become more sinuous, or winding. She said most submarine channels on continental slopes are inactive.
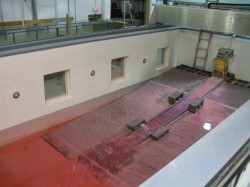
“Where these systems are active, the turbidity currents going through them are so energetic and destructive that studying them is very difficult.” she said. “So, we need to get innovative to address questions related to turbidity current activity and their interaction with seafloor topography.”
She said seismic data from ancient submarine channels and stratigraphic analysis of outcrops can offer insights, but to study the ongoing evolution of channels, the UTML water tank is essential.
Aymeric Peyret is a doctoral student working under the supervision of David Mohrig. He uses computer models to try to replicate the results of water tank experiments and so gain a better understanding of the physics that underlies turbidity currents. He said scientists don’t know why some turbidity currents erode channels in the seafloor while others fill them in. Factors might include the initial conditions (such as seafloor topography and how cohesive the sediments are), the density ratio between the turbidity current and the surrounding water and how fast the current is when it enters the water.
“Finding where the transition between erosion and deposition lies would be a nice holy grail to discover,” he said.
Bigger is Better
In January 2009, the team ran an experiment for several days and began to see something that neither they nor anyone else in their field had ever observed before: their sediment-laden turbidity current formed channels in the existing sediment. It was a big step closer to creating a true submarine landscape in the lab.
“When we saw that our jaws dropped,” said Buttles.
This early success makes them confident that their tank is doing a reasonable job of modeling some aspects of real turbidity currents and ultimately, seascape evolution.
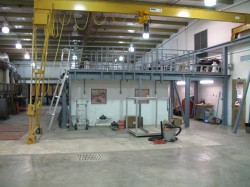
So why were they more successful with the new tank?
It comes down to size. The new tank is basically a larger version of their MIT system. The increased depth allows the researchers to create a relatively steep sloping platform and the larger holding capacity means they can run experiments longer.
“Both of these conditions are key to our recent success,” said Buttles.
The steep sloping platform, he explained introduces a greater driving force due to gravity for the turbidity currents (relative to the MIT setup), “which translates into a greater potential to erode and transport our sediment. Sediment bed changes may evolve at a slow rate and can be quite subtle, the longer runtime allows the evolution to proceed under constant experiment conditions.”
For Mohrig and Buttles, this means they finally have a chance to answer some of the fundamental questions about turbidity currents that have stymied scientists for decades.
by Marc Airhart
For more information about the Jackson School contact J.B. Bird at jbird@jsg.utexas.edu, 512-232-9623.