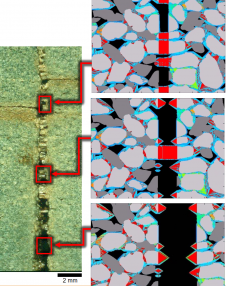
Figure 1 A crack tip in tight gas sandstone showing quartz deposits (highlighted by boxes) and three simulations of synkinematic quartz accumulation from Lander & Laubach (2015).
Deformation mechanisms are the various processes, usually at the grain scale or smaller, that are responsible for accommodating penetrative strains in rocks.
Under the low temperature (less than 300℃) conditions of sedimentary basins, grain-scale deformation processes are a part of diagenesis, the microstructural and chemical changes that turn sediments into rock and that through time modify rock properties. Although traditional diagenesis studies tend to neglect all but the earliest post-depositional deformation mechanisms, penetrative processes at greater depth such as solution-precipitation creep, stress corrosion, and crystal plasticity can have a profound impact on rock properties as well as influencing the development of large-sized fractures.
The chemical aspects of deformation mechanisms associated with fracture growth have been relatively neglected. But as illustrated in figure 1, evidence of chemical processes are to be found in key areas such as crack tips, where profound influences on fracture growth are possible.
An example of an important deformation mechanism is solution-precipitation creep, which is widely attested to affect fracture host rocks and has been implicated to cause fracture growth to deviate from what is predicted by linear elastic fracture mechanics.
Solution-precipitation creep and how it might influence fracture growth is the focus of my research.
As illustrated in figure 2, solution-precipitation creep, sometimes referred to as diffusive mass transfer (although strictly speaking, these terms are not equivalent), is a three-stage process including
- solution of material at a source,
- movement (advection or diffusion) of the material along some mass transfer pathway, and
- precipitation of material at a growth site (a sink).
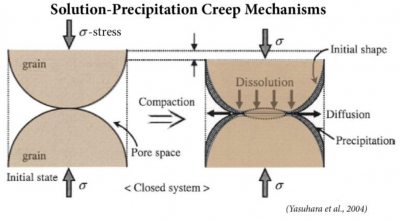
Figure 2 Mechanisms of solution-precipitation creep recognized in fracture host rocks.
The process is widespread and is likely to interact with fracture growth. Evidence of solution-precipitation creep has been found in fault rocks and elsewhere as documented in the Further Reading listed below.
Little is known, however, about how solution-precipitation creep might modify the size and patterns of millimeter- to meter-sized or larger opening-mode fractures. Yet the sizes and patterns of such fractures exert fundamental controls on fluid flow and strength of deeply buried rocks.
Creep and large-sized fractures
Obvious ways that solution-precipitation creep can modify how fractures grow is by altering host rock mechanical properties or by dissipating driving stresses. But my interest is in how solution-precipitation creep can modify the process of fracture tip advance.
My work follows up field and petrographic studies by Peter Eichhubl and Atilla Aydin (2003) who documented opening-mode fractures in near-surface mudstones that had experienced rapid in situ hydrocarbon combustion, turning the mudstones into a residue called clinker. These unusual rocks are possibly useful guides for the subtler, but more widespread, and probably slower processes that may affect deep-seated rocks. As shown in figure 3, near the fracture tip that study found arrays of elongated pores with evidence of pore coalescence.
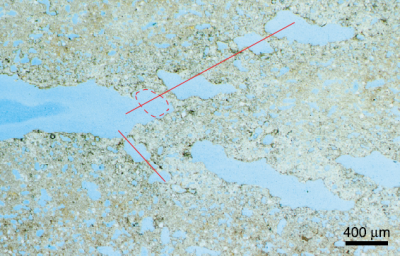
Figure 3 Elongated pores ahead of a blunt fracture tip, transmitted light image. From Eichhubl & Aydin (2004).
The inference drawn from these patterns is that the elongated pores originated by a process of solution-precipitation creep that may have been associated with inelastic deformation, which they believed could control fracture initiation, propagation, and final shape.
An example is shown in Figure 3; the fracture traces are marked by red lines. Blue shows porosity.
I found similar textures in my Master’s research, in fractures from the Campito Formation, in eastern California. These fractures formed under temperatures between 150°C and 250°C, and have elliptical aperture profiles with blunting of the tip. Fractures that formed under temperatures higher than 300°C, on the other hand, have triangular shapes. I concluded that the triangular aperture profiles may result from incremental elastic fracture growth alternating with stages of stress dissipation by solution-precipitation creep, while the tip blunting may result from enhanced solution-precipitation creep in the vicinity of fracture tips.
Evidence of the possible role of non-elastic processes and solution-precipitation creep also comes from standard reservoir rocks sampled with deep cores from East Texas. Based on fracture shapes and evidence of fracture shape through time derived from the patterns of crack-seal cement layer thickness and number of fracture opening increments on samples, Alzayer et al. (2015) found evidence of non-linear fracture growth kinematics. Fracture growth happened into two stages. In the first stage, the fracture grew faster in length than in width or aperture. During the second stage, the fracture grew faster in aperture than in length. This widening pattern could result from fracture-opening strain accommodated by solution-precipitation creep and concurrent slow, possibly subcritical, fracture growth.
Solution-precipitation creep:
what is it?The driving force for solution-precipitation creep is a chemical potential gradient, or the stress difference in chemical potential of the solid between the dissolution zone and the deposition zone. The degree of the gradient is influenced by multiple factors such as local stress variations (Rutter, 1983; Wheeler 1987), chemical and fluid pressure gradients (Etheridge et al. 1984), and the internal strain energy of individual grains (Wintsch and Dunning, 1985).
Possible transport mechanisms of the source material along some pathways are diffusion in an aqueous pore fluid, lattice or bulk diffusion, surface diffusion, vapor phase transport, grain boundary diffusion, and advection due to the fluid that flows through fractures or along grain boundaries (Knipe, 1989; Eichhubl and Aydin, 2003; Oliver and Bons, 2001).
Evidence of solution-precipitation creep includes blunt fractures, certain cement deposits, and stylolites.
Caution!
So far, I have only talked about solution-precipitation creep as if it were the sole deformation mechanism in rock that might affect fracture growth. But in nature, solution-precipitation creep usually operates in conjunction with other mechanisms that can either facilitate or hinder fracture growth.
An example is the force of crystallization, a process in which fracture cement that grows into fracture walls under certain circumstances may exert forces large enough to initiate fracture growth. The process is controversial, but among the papers that have advocated for it are Wiltschko and Morse (2001), Noiriel et al. (2010), Li et al. (2016), and Meng et al. (2018).
On the other hand, microfractures in fracture tip regions may be filled by precipitation processes as in figure 1, which may slow down or stop fracture growth. Work by Hooker et al. (2012) has highlighted the likely importance of this process. When a fracture tip is blunted or rounded due to solution-precipitation creep, the fracture may not extend because of insufficient stress concentration (Fischer-Cripps, 2007). The presence of a gradient of solubility in a small fracture can lead to dissolution from the lateral walls and precipitation at the fracture tip, which then enhances the tip blunting further (Ito and Tomozawa 1982; Ciccotti, 2009) thus hindering further growth.
Fracture cement can also increase fracture stiffness by resisting fracture closure and re-opening, processes that are suspected to possibly influence clustering and fracture size distributions (Laubach et al., 2004; Laubach and Ward, 2006; Olson et al., 2007; Olson et al., 2009; Hooker et al., 2018).
Next steps
Overall, various observations are compatible with solution-precipitation creep being an important but underappreciated deformation mechanism affecting fracture growth in moderately to deeply buried sedimentary rocks. The process can cause inelastic deformation that results in fracture attributes that deviate from what is predicted by linear elastic fracture mechanics such as non-elliptical shape or blunt tips, which in turn could affect how entire fracture patterns develop. Together with other deformation mechanisms, a study of solution-precipitation creep is a useful path for investigation fracture growth at depth.
My experimental tests and field work on this topic are underway. Look forward to reports on my progress.
By Natchanan (Mint) Doungkaew, a graduate student studying chemical and mechanical interaction in fracture growth.
Alzayer, Y., Eichhubl, P., and Laubach, S.E., 2015, Non-linear growth kinematics of opening-mode fractures: Journal of Structural Geology, 74, 31–44, doi: 10.1016/j.jsg.2015.02.003. | view at publisher
Ciccotti, M., 2009, Stress-corrosion mechanisms in silicate glasses: Journal of Physics D: Applied Physics, 42, no. 21, 214006.
Eichhubl, P., and Aydin, A., 2003, Ductile opening-mode fracture by pore growth and coalescence during combustion alteration of siliceous mudstone: Journal of Structural Geology, 25, no. 1, 121-134.
Etheridge, M. A., Wall, V. J., Cox, S. F., and Vernon, R. H., 1984, High fluid pressures during regional metamorphism and deformation: Implications for mass transport and deformation mechanisms: Journal of Geophysical Research: Solid Earth, 89, no. B6, 4344-4358.
Fischer-Cripps, A. C., 2007, Introduction to contact mechanics, Springer. p. 35
Hooker, J. N., Laubach, S. E., and Marrett, R., 2018, Microfracture spacing distributions and the evolution of fracture patterns in sandstones: Journal of Structural Geology, 108, 66-79. | view at publisher
Hooker, J.N., Gomez, L.A., Laubach, S.E., Gale, J.F.W. & Marrett, R., 2012, Effects of diagenesis (cement precipitation) during fracture opening on fracture aperture-size scaling in carbonate rocks. In Garland, J., J.E. Neilson, S.E. Laubach, & K. J. Whidden (eds.) Advances in Carbonate Exploration and Reservoir Analysis, Geological Society of London Special Publications 370, 187-206. doi:10.1144/SP370.9. | view at publisher
Ito, S., and Tomozawa, M., 1982, Fracture Blunting of High-Silica Glass: Journal of the American Ceramic Society, 65, no. 8, 368-371.
Knipe, R. J., 1989, Deformation mechanisms — recognition from natural tectonites: Journal of Structural Geology, 11, no. 1, 127-146.
Lloyd, G. E., and Knipe, R. J., 1992, Deformation mechanisms accommodating faulting of quartzite under upper crustal conditions: Journal of Structural Geology, 14, no. 2, 127-143.
Lander R.H., and Laubach, S.E., 2015. Insights into rates of fracture growth and sealing from a model for quartz cementation in fractured sandstones. Geological Society of America Bulletin 127 (3-4), 516-538. doi: 10.1130/B31092.1 | view at publisher | blog post
Laubach, S. E., Olson, J. E., and Gale, J. F. W., 2004, Are open fractures necessarily aligned with maximum horizontal stress?: Earth and Planetary Science Letters, 222, no. 1, 191-195. | view at publisher
Laubach, S.E., and Ward, M.E., 2006, Diagenesis in porosity evolution of opening-mode fractures, Middle Triassic to Lower Jurassic La Boca Formation, NE Mexico: Tectonophysics, 419, no. 1, 75-97. | view at publisher
Laubach, S. E., Eichhubl, P., Hilgers, C., and Lander, R. H., 2010, Structural diagenesis: Journal of Structural Geology, 32, no. 12, 1866-1872. | view at publisher
Li, S., Suo, Y., Yu, S., Wu, T., Somerville, I., Sager, W., Li, X., Hui, G., Zhang, Y., Zang, Y., and Zheng, Q., 2016, Orientation of joints and arrangement of solid inclusions in fibrous veins in the Shatsky Rise, NW Pacific: implications for crack‐seal mechanisms and stress fields: Geological Journal, 51, no. S1, 562-578.
Meng, Q., Hooker, J., and Cartwright, J., 2018, Displacive widening of calcite veins in shale: insights into the force of crystallization. Journal of Sedimentary Research 88 (3), 327-343.
Noiriel, C., Renard, F., Doan, M.-L., and Gratier, J.-P., 2010, Intense fracturing and fracture sealing induced by mineral growth in porous rocks: Chemical Geology, 269, no. 3, 197-209.
Oliver, N. H. S., and Bons, P. D., 2001, Mechanisms of fluid flow and fluid–rock interaction in fossil metamorphic hydrothermal systems inferred from vein–wallrock patterns, geometry and microstructure: Geofluids, 1, no. 2, 137-162.
Olson, J. E., Laubach, S. E., and Lander, R. H., 2007, Combining diagenesis and mechanics to quantify fracture aperture distributions and fracture pattern permeability: Geological Society, London, Special Publications, 270, no. 1, 101-116. | view at publisher
Olson, J. E., Laubach, S. E., and Lander, R. H., 2009, Natural fracture characterization in tight gas sandstones: Integrating mechanics and diagenesis: AAPG Bulletin, 93, no. 11, 1535-1549. | view at publisher
Passchier, C. W., and Trouw, R. A. J., 2005, Microtectonics, New York; Berlin Springer.
Rutter, E. H., 1983, Pressure solution in nature, theory and experiment: Journal of the Geological Society, 140, no. 5, 725-740.
Wheeler, J., 1987, The significance of grain-scale stresses in the kinetics of metamorphism: Contributions to Mineralogy and Petrology, 97, no. 3, 397-404.
Wiltschko, D. V., and Morse, J. W., 2001, Crystallization pressure versus “crack seal” as the mechanism for banded veins: Geology, 29, no. 1, 79.
Wintsch, R. P., and Dunning, J., 1985, The effect of dislocation density on the aqueous solubility of quartz and some geologic implications: A theoretical approach: Journal of Geophysical Research: Solid Earth, 90, no. B5, 3649-3657.
Yasuhara, H., Elsworth, D., and Polak, A., 2004, Compaction and Diagenesis of Sandstones – the Role of Pressure Solution, in Stephanson, O., ed., Elsevier Geo-Engineering Book Series, Volume 2, Elsevier, 733-738.
© 2025 Jackson School of Geosciences, The University of Texas at Austin