Research
Students interested in working with me are welcome to email me, to discuss possible projects broadly related to these topics–I like talking about my work!
Lithologic and Climatic Controls on Landscapes
While the control of lithology on topography is apparent in many landscapes, there is a significant lack of quantitative, mechanistic or predictive understanding of how measurable rock properties influence landscape form and evolution. If functional relationships among topography, erodibility, weatherability and rock properties were better understood, it should be possible to put imperfect but quantitative bounds on relative rock properties from topographic data alone—i.e. “reading” lithology from topography. Currently this cannot be done across a landscape to any degree of certainty.
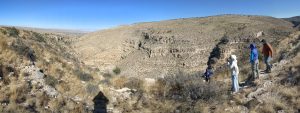
Hiking out of a Canyon in the Guadalupe Mtns. Lithologic control of hillslope form is apparent across the valley.
PhD student Grace Guryan is working to understand how lithology controls landform evolution, using the Guadalupe Mountains of Texas and New Mexico as a field site. This NSF-supported project is a collaboration with Dr. Nicole Gasparini at Tulane University, and several other people. Bedrock properties control not only the local erodibility of the bed, but also coarse sediment production that represents erosion of the upstream watershed. These dual roles of lithology result in interesting feedbacks and make relations with topographic evolution nonunique, and therefore interesting. A key limitation of current landscape evolution models is how to integrate measurable rock properties (strength, bed thickness, fracture spacing and orientation) into erodibility parameters. By combining field measurements, available high-resolution topography (airborne Lidar) and landscape evolution modeling using LandLab, we are evaluating how bedrock properties control hillslope and channel form. Tulane PhD student Sam Anderson recently published a study of boulder and bedrock relations with channel form in the Guadalupe Mountains.
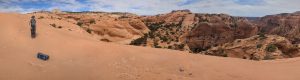
A tributary to French Spring Fork of Happy Canyon, Utah.
Improving methods for inferring relative rock property differences from topography also holds great potential for understanding planetary surface geology. PhD student Morgan Carrington, coadvised by Dr. Tim Goudge, is exploring how not only surface runoff vs. groundwater flow but also lithologic contrasts control the morphology of canyon headwalls on both Mars and the Colorado Plateau (in Southern Utah). Earth is far from a perfect analogue to Mars, and understanding how controls are different as well as similar is important to comparative planetology.
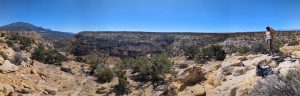
Tarantula Mesa, Utah
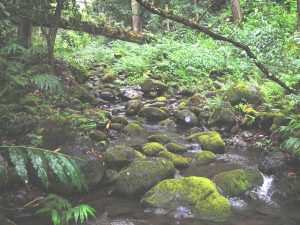
Wet side of Kohala Peninsula, with basalt boulders.
What are the mechanisms by which climate influences erosion rates? Landscape erosion influences global climate because chemical weathering of silicate minerals consumes atmospheric CO2. Topography affects climate regionally and even globally by influencing atmospheric circulation patterns. Importantly, numerical models suggest that erosion may be able to localize isostatic rock uplift and tectonic deformation. If local erosion rates are sensitive to local climate, then climate may control the tectonic and topographic evolution of mountain ranges. However, field studies have found conflicting results on the strength of empirical correlations between climate and erosion. A key limitation in our ability to interpret and predict these feedbacks is understanding how strongly, and by what mechanisms, climate actually influences erosion rates.
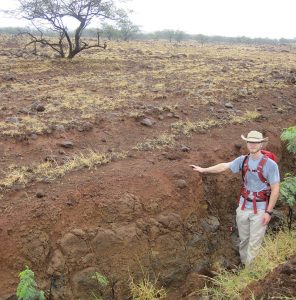
Note the regolith weathering profile. Brendan Murphy on the dry side of Kohala Peninsula, Hawaii.
To address these questions, former PhD student Dr. Brendan Murphy studied how patterns of incision relate to climate across the Kohala Peninsula of Hawai’i. This work was done in collaboration with Dr. Nicole Gasparini at Tulane University, and several other people. In this landscape, we found that the key mechanism of climatic control of incision was chemical weathering of basalt, which made the bedrock physically weaker and more erodible. While chemical weathering of the rock surface increases erodibility, erosion removes this surficially weathered bedrock, exposing fresher and less erodible rock beneath (Murphy et al., 2016, 2018). Thus, erodibility is influenced by both climate and erosion rate. We have developed new equations to explicitly model feedbacks among bedrock erodibility, erosion rate, the degree of chemical weathering, and chemical weathering rate. As part of this project, Tulane PhD student Dr. Jianwei Han focused on landscape evolution modeling of how climate gradients influence basin evolution (Han et al., 2014, 2015).
Mountain River Morphodynamics
Robust morphodynamic models are needed to predict how rivers may respond to environmental perturbations such as floods, changing climate, or river restoration projects. River feedbacks also influence landscape evolution over longer timescales through downcutting and lateral migration. Research in my group addresses river morphodynamics in a variety of ways.
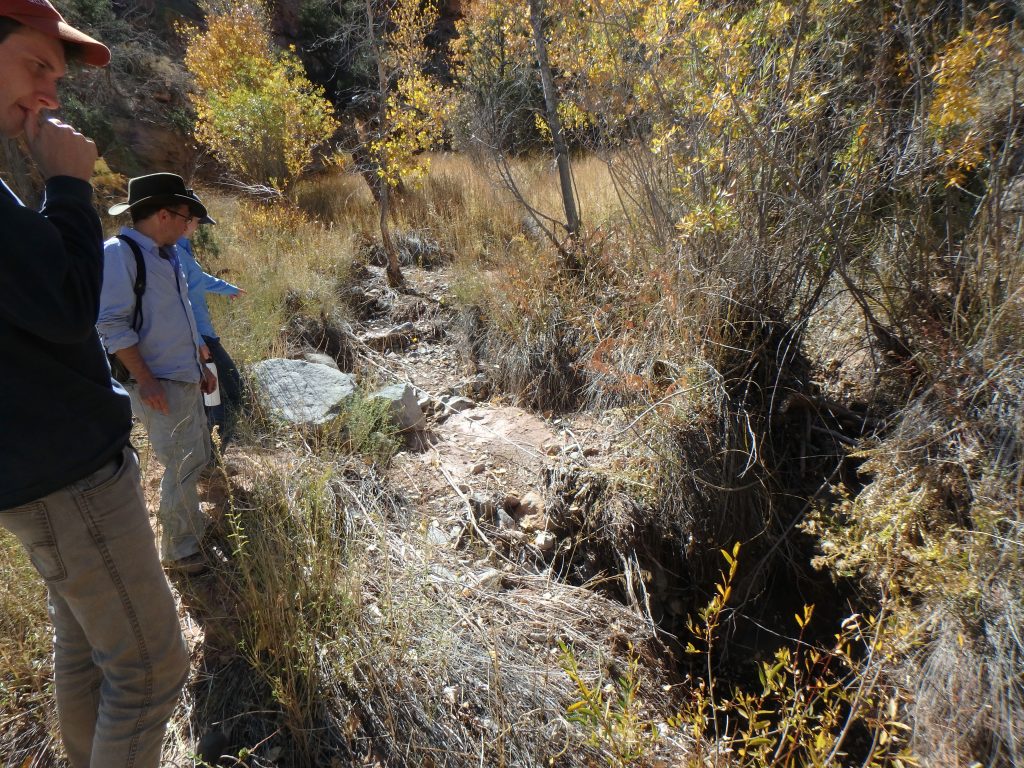
Paul ponders vegetation in the channel, with Dr. Wayne Baumgardner (NASA) and Dr. Ashley Matheney.
Former MS student Paul Southard explored how vegetation influences channel morphology, published in WRR. This work took advantage of a unique field site—lithologically controlled springs/seeps along channels in the Henry Mountains of Utah. The key opportunity provided by this site is that the same channels go from essentially unvegetated to vegetated and back to unvegetated as one goes downstream. Paul used a combination of airborne and ground-based LiDAR processing and ANUGA flow modeling to evaluate how vegetation influences morphology and flow. This research is being done in collaboration with UT colleagues Dr. Daniella Rempe and Dr. Ashley Matheney, with more to come.
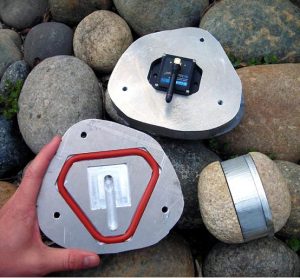
These are the very first smartrocks I machined, from 2008.
Improving bedload transport predictions during floods is one key to understanding channel morphodynamics. Bedload transport is inherently stochastic and probabilistic. Recent work using insights from statistical physics has suggested that bedload transport exhibits anomalous dispersion and nonlocality. Former PhD students Dr. Lindsay Olinde and Dr. Kealie Pretzlav both used “smartrocks” (tracer cobbles instrumented with accelerometers) to quantify bedload transport statistics during natural snowmelt floods. Lindsay also used “better than average rocks” (RFID-tagged clasts) (Olinde and Johnson, 2015; Underwood, 2012). We are continuing to explore rest time and hop time distributions and what their scaling says about anomalous diffusion. We are also using smartrocks to measure changes in thresholds of motion during floods (Pretzlav et al., 2020; Pretzlav et al., 2021).
We also use flume experiments to quantify and understand a variety of feedbacks, including channel responses to pulses of fine gravel (Johnson et al., 2015), and the influence of coarse grain clustering on bed stability (Johnson, 2017). One direction I am particularly excited about is understanding how “thresholds” of gravel motion evolve in streams as a function of the history of both sediment supply (Johnson, 2016) and discharge. I am now working on this problem in collaboration with a variety of people including Dr. Claire Masteller at Washington University in St. Louis.
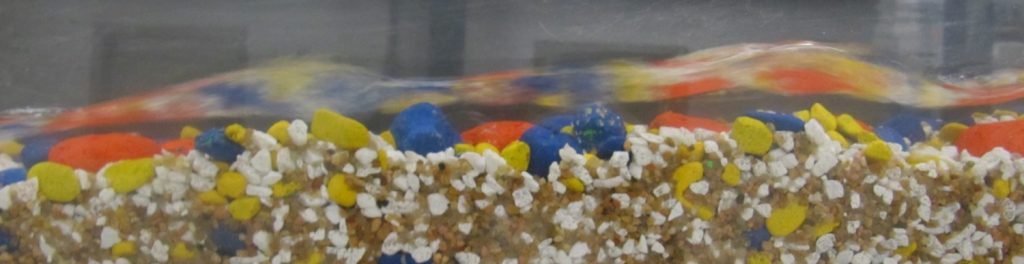
Side view of flume; blue grains are ~16 mm in intermediate diameter.
From Sediment Deposits to Flow Hydraulics
Tsunamis and hurricane storm surges are both devastating and very rare at any given location. Historical records are usually too short to accurately assess risk. Because sediment can imperfectly record these extreme events, in principle we should be able to quantitatively “read” histories of events from deposits. Relatively simple models using suspended sediment transport relations have been proposed to calculate wave depths and velocities from deposit grain size distributions, but have not been rigorously evaluated with independent data. Validated and improved paleohydraulic inverse models are needed to determine coastal hazards. Equally important is putting rigorous uncertainty bounds on these interpretations, as flow depth and velocity are inherently under-constrained by sediment deposits.
In collaboration with my colleagues Dr. Wonsuck Kim and Dr. David Mohrig and former UT Masters student Katie Delbecq, we conducted scaled tsunami experiments of sediment entrainment, transport in suspension, and deposition. The experiments were conducted in a unique laboratory flume I developed at UT, which uses a computer-controlled lift gate to instantaneously release ~ 6 m3 of water. We show that a disequilibrium model that accounts for advection and settling of grains can predict experimental flow depths to roughly a factor of two (Johnson et al., 2016). Prediction uncertainties were also rigorously quantified. Johnson et al. (2017) show that depth and velocity predictions can be substantially improved by modifying the existing model to take into account turbulent dispersion of grains.
Much of our predictive understanding of sediment transport assumes steady uniform flow, but a significant fraction of Earth’s subaerial surface is in dryland landscapes, with channels that are usually not flowing. We have also conducted flume experiments looking at flash flood hydraulics and bedload transport, with Dr. Suresh Kumar Thapetta (now at VNIT Nagpur), Dr. Jonathan Laronne at Ben-Gurion University of the Negev, and others. Here is a paper comparing methods for calculating shear stresses during bores and rapidly changing hydrographs. Also a video of one of our experiments put together by undergrad research assistant Rebecca Rhodes.
Thanks for reading!