Proposal
Executive Summary/Abstract
Rock-fluid interaction experiments are widely used in both academic and industrial applications, where researchers aim to address challenges such as oil-water contacts in the oil and gas industry, as well as applications like transport flow modeling in scientific institutions.
Water samples generated from this study’s experimental setup will be analyzed using solution-mode ICP-MS to determine their elemental composition and assess the impact of gas-rock interactions on the collected water samples. This project aims to develop a solution-mode ICP-MS method to analyze chemical composition changes in water produced from hydrogen/nitrogen rock-fluid interaction experiments. Ultimately, the results will help outline the impact of hydrogen reactivity with rocks and their potential for hydrogen storage.
Research Objective
This study aims to analyze deionized (DI) water generated from a rock/fluid interaction in an autoclave experiment under high pressures and temperatures. The elemental analysis of water generated from the reactivity of each gas (hydrogen and nitrogen) is planned to be quantified using ICP-MS solution mode, where the precise elemental composition of the water will reveal any chemical reactions occurring and dissolution of the rock and its behavior with different gases and with no gases. Several elements are planned to be measured including Li, B, Na, Mg, Al, Si, P, K, Ca, Ti, V, Cr, Mn, Fe, Ni, Cu, Zn, Se, Rb, Sr, Mo, Ru, Rh, Pd, Sb, Ba, Ir, Pt, Pb, and U. The selection of analytes based is on typical elements analyzed in water samples for mineral content (e.g., Li, B, Na, Mg, K, Ca) (Lu et al., 2017), metals (e.g., Mn, Cu, Zn, Pb) (Kuznetsova et al., 2019), mineral dissolution phases in sandstone/feldspar weathering or hematite dissolution (e.g., Na, Al, Si, S, K, Ca, Fe) (Kaszuba et al., 2013), and investigation of contaminants or catalysts from autoclave reaction vessels (e.g., Ce, Ni, Ru, Rh, Pd, Ir, Pt) (Truche et al., 2013; Yekta et al., 2018). All of the analytes mentioned above can be incorporated after running the “Full Spectral Mass Scan” on the ICP-MS.
Significance/Justification
The method provides a foundation for conducting similar autoclave experiments aimed at investigating rock-fluid interactions in a closed, gas-charged system. Monitoring chemical changes in water composition can aid in modeling the kinetics of energy resource generation or storage, including hydrogen. Elemental composition changes are especially crucial in sandstone dissolution reactions, as demonstrated in this experimental setup, which can help evaluate the potential of the rock as a viable candidate for hydrogen storage.
Literature Review
Numerous autoclave experiments in the literature involve reacting different materials and using various instruments to assess changes in chemical composition.
In the study by Hassanpouryouzband et al. (2022), the geochemical reactivity of hydrogen with sandstone reservoirs was investigated to model hydrogen storage potential in these reservoirs. The experimental setup used an autoclave-like apparatus, but the elemental analysis of the generated water was limited to the following analytes: Ag, Al, Ba, Ca, Cd, Ce, Co, Cr, Cu, Fe, Hg, K, Mg, Mn, Na, Ni, P, Pb, S, Si, Sr, Ti, Zn, and Zr. This limitation was due to the use of ICP-OES, which has lower detection capabilities than the more sensitive ICP-MS.”
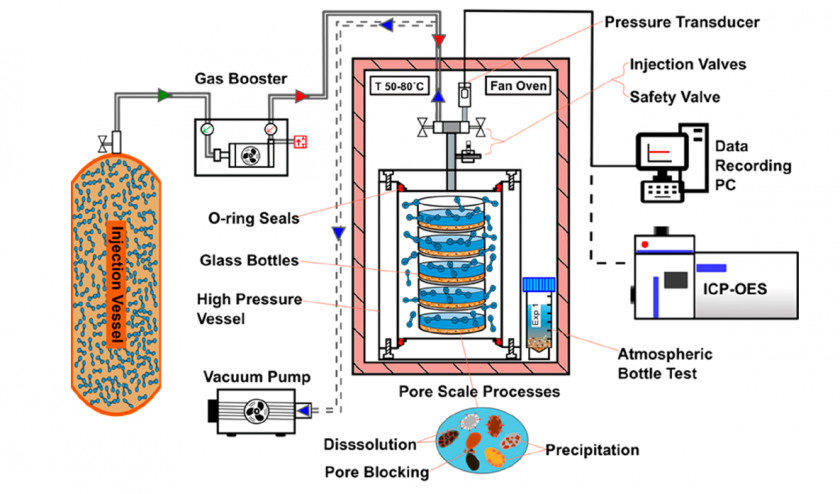
Additionally, in the study by Lu et al. (2017), a similar experimental setup was established using an autoclave reaction vessel to investigate geochemical interactions between Hosston sandstone and Eagle Ford brine through reaction experiments and geochemical modeling. Furthermore, SEM observations along with Mercury Injection Capillary Pressure (MICP) data were used to distinguish between reacted and unreacted grains. The results concluded that the overall effects of rock-water reactions on rock properties were minimal.
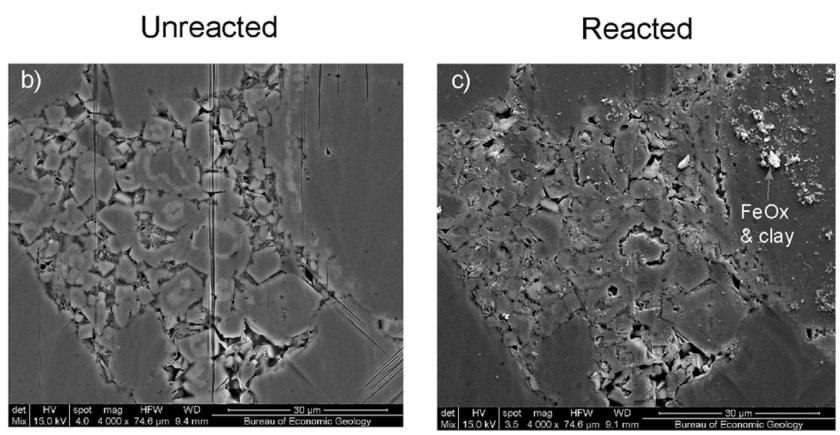
Several analytical challenges may arise, including interferences and matrix effects, such as those from the dissolution of feldspars and clay minerals in sandstone samples. Based on a literature review, particularly the work by Lu et al. (2017), the matrix effect was studied using SEM observations of the analyzed samples, which allowed for correlating the effect of feldspar and clay dissolution on elemental composition.
Challenges in determining rare earth element (REE) concentrations in water samples via ICP-MS analyses include interferences, such as isobaric interferences (e.g., between 142Ce and 142Nd, 174Yb, and 174Hf). Additionally, polyatomic interferences—such as those from metal oxides, hydroxides, hydrides, chlorides, and argides—are even more problematic than isobaric interferences, as they are more difficult to correct. These interferences depend on both the abundance of at least two isotopes and the rate of molecule formation (Wysocka, 2021).
Furthermore, a powerful technique for mitigating spectral interferences of elements was discussed by Rowan and Houk (1989), in which several gases (Xe, He, Ar, CH4) were used to reduce polyatomic ion interferences. For example, interferences for As and Se, which overlap with 40Ar35Cl and 40Ar37Cl, respectively (May & Wiedmeyer, 1998), can be mitigated by using collision reaction cells (CRC) with appropriate collision gases.
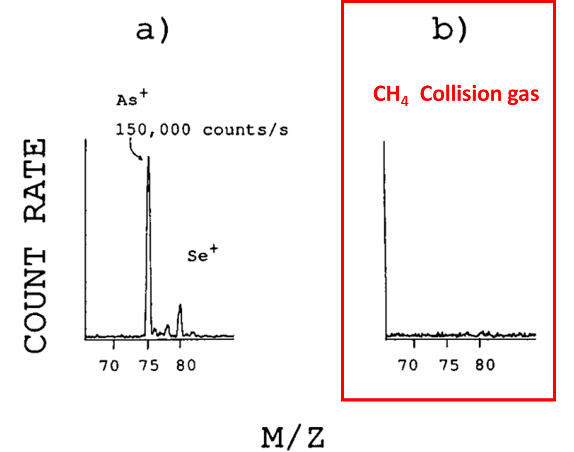
Materials and Methods
The project involves analyzing water samples generated from rock-fluid interaction autoclave experiments. Seventeen water samples are expected to undergo ICP-MS elemental analysis. As part of sample preparation, the water samples will be acidified using high-purity nitric acid specifically designed for trace element analysis (trace metal grade HNO3). Based on the sample Total Dissolved Solids (TDS), which should be less than 200 ppm, an appropriate dilution will be determined if necessary, and a dilution factor (DF) will be calculated accordingly.
Additionally, common internal standards will be used in the ICP-MS sample analysis to cover the analytical mass range, including beryllium (9Be), scandium (45Sc), germanium (72Ge), indium (115In), tellurium (125Te), and lutetium (175Lu). For trace metal analysis in water, NIST quality control (QC) calibration commercial standard NIST-1643f will be used, as Beauchamp et al. (2020) recommended.
Furthermore, a method can be established for analyzing the sample set after preparing calibration and quality check standards. An important aspect of method setup is selecting the appropriate Collision Reaction Cell (CRC) mode, which commonly includes He, H2, and no-gas modes; He mode is typically used to handle polyatomic interferences. Alternatively, a Dynamic Reaction Cell (DRC) can be used for more complex acid matrices, such as phosphoric and sulfuric acids (Kawabata, Kishi, & Thomas, 2003)
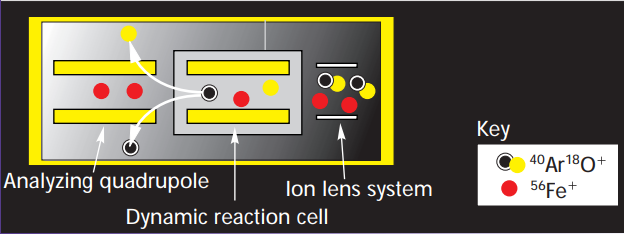
Possible Outcomes
The method would set the stage for integrating water analysis results into the rock/fluids interactions model where possible kinetics changes can be modeled to tell a story on the gases interacted with the rocks and their potential in terms of generation or storage as an energy source. Additionally, the method may indicate scenarios of outcomes related to the reaction of the rocks with gases injected into an autoclave closed system, and these scenarios may lead to preliminary conclusions of the effect of mineral dissolution from the rocks interacted.
Timeframe and Budget
For a timeframe, the seventeen water samples, including preparations that mainly involve acidification of the samples, running calibration standards, blanks, and quality check (QC) standards, along with picking elements and isotopes to be analyzed for this solution mode run, should be automatic within a day and obtained results from unattended operation overnight.
For the budget, given that solution mode costs $20 per analysis and we have 17 water samples to be analyzed, which should have calibration, quality control, and blank analyses, adding between 23-26 analyses and leaving us between 38-41 analyses. Thus, the total cost of the sample analyzed would be between $760-820, inclusive of all calibration standards, quality control standards, blanks, spikes, and unknowns.